Gravitational-Wave Instrumentation
Context: The direct detection of gravitational waves (GWs) is a breakthrough discovery of recent years. The several GW detections by the Advanced LIGO and Virgo detectors, since they were first discovered in 2015, have opened up a new window to the observable universe. The current “second generation” detectors are Michelson interferometers with km-scale arms. The principle behind the detector is based on the fact that when a GW passes through, the arms of the interferometer are stretched and squeezed in opposite ways in the two directions.
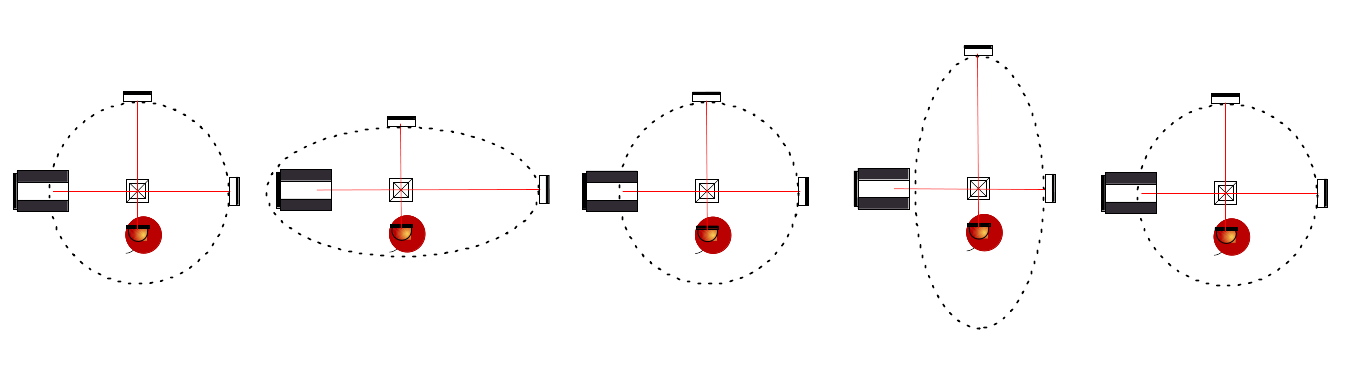
The current detectors will eventually be replaced by the next generation of GW detectors of significantly higher sensitivity and distance reach. One of these “third generation” detectors is the Einstein Telescope (ET), which is planned to be built in Europe in the 2030s. Fundamentally new technology will be required to go beyond the limitations of the current detectors. Extensive work and study is required for each of the new techniques to be implemented. ETpathfinder is an R&D facility, located in Maastricht, the Netherlands, with the aim to test these new techniques and to give important inputs for the design and the construction of third generation GW detectors like ET. The ETpathfinder is a Fabry-Perot Michelson interferometer with 10 m arm cavities working at cryogenic temperatures. It will mainly focus on developing prototypes and testing cryogenic temperatures (120 K, 15 K), new mirror material (Silicon), new laser wavelengths (1550 nm, 2090 nm), and advanced quantum noise reduction techniques.
Project 1: Contribution to the development of ETpathfinder
Ghent University has been involved in the ETpathfinder project since its beginning. The facility is currently under construction, with the aim to have the interferometer up and running by the end of this year.
An R&D research facility for optical characterization at 2µm will be set up in the next months at Campus Proeftuin in Ghent, where we will develop components for installation at the facility in Maastricht.
The student will be actively involved in the development of ETpathfinder, with a project that will be chosen according to the experimental skills and the schedule of the project.
Depending on the expertise and interest of the student, the possible topics are:
- Optical simulations
- Optical characterization
- Assembly and commissioning of optomechanical devices
The student will be a member of the ET Collaboration. He/she would get involved with the technology and challenges of interferometric detectors in the active field of gravitational-wave science. The project will be done in collaboration with the ETpathfinder community. Travel to Maastricht, where the facility is located, may be required.
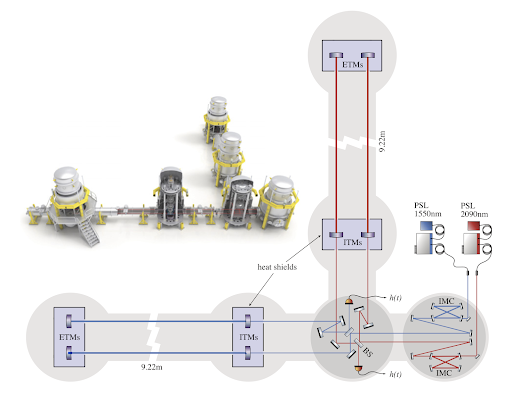
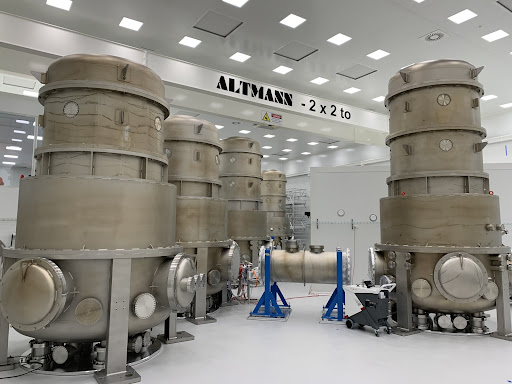
Contact: Dr. Daniela Pascucci
Project 2: Data analysis of the Phase Cameras of Advanced Virgo
Phase Cameras are powerful sensors that can help to improve the sensitivity of gravitational waves detectors. They can be used to help the commissioning activity and to implement feedback to correct the thermal effect that creates aberrations of the mirrors.
In the current Advanced Virgo configuration two Phase Cameras are present: one after the Power Recycling mirror and one at the dark port of the interferometer (see figure). However, we are not using Phase Cameras to their full potential. So far in fact only the intensity of the wavefront is studied to determine the higher-order-mode content, and the phase cameras are only used as a monitor of the wavefront aberrations. We still do not know how to use the phase information and no feedback is implemented to correct the mirror’s aberrations.
The student will work on the development of a script that allows analysing the phase cameras data using both the intensity and the phase information and to study how to use this information to implement feedback.
Basic knowledge of optics and interferometry and a knowledge of Matlab and Python is required.
The student will be a member of the Virgo Collaboration. He/she would get involved with the technology and challenges of interferometric detectors in the active field of gravitational-wave science.
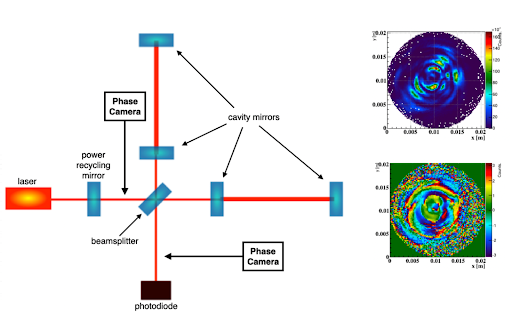
Contact: Dr. Daniela Pascucci
Gravitational-Wave Data Analysis
Context: The direct detection of gravitational waves (GWs) is a breakthrough discovery of recent years. The 2015-discovery has opened a new window to the observable universe. Over the subsequent observing runs, the GW detector network, comprising the LIGO, Virgo, and KAGRA detectors, has observed numerous coalescences of compact binaries — binaries of black holes and neutron stars — and has given us a wealth of novel results in fundamental physics, astrophysics, and cosmology. The detector network is currently undergoing upgrades, and a fourth observing run is expected to start in May 2023. The detector network is going to further expand and increase in sensitivity over the subsequent years and will eventually be replaced by the next generation of detectors of much higher sensitivity and distance reach. The proposed and planned “third generation” GW detectors include the Cosmic Explorer (CE) and the Einstein Telescope (ET). With upgrades, expansion, and new detectors, we will not only come across many more similar observations, but also detections of different kinds, pushing the current boundaries of physics.
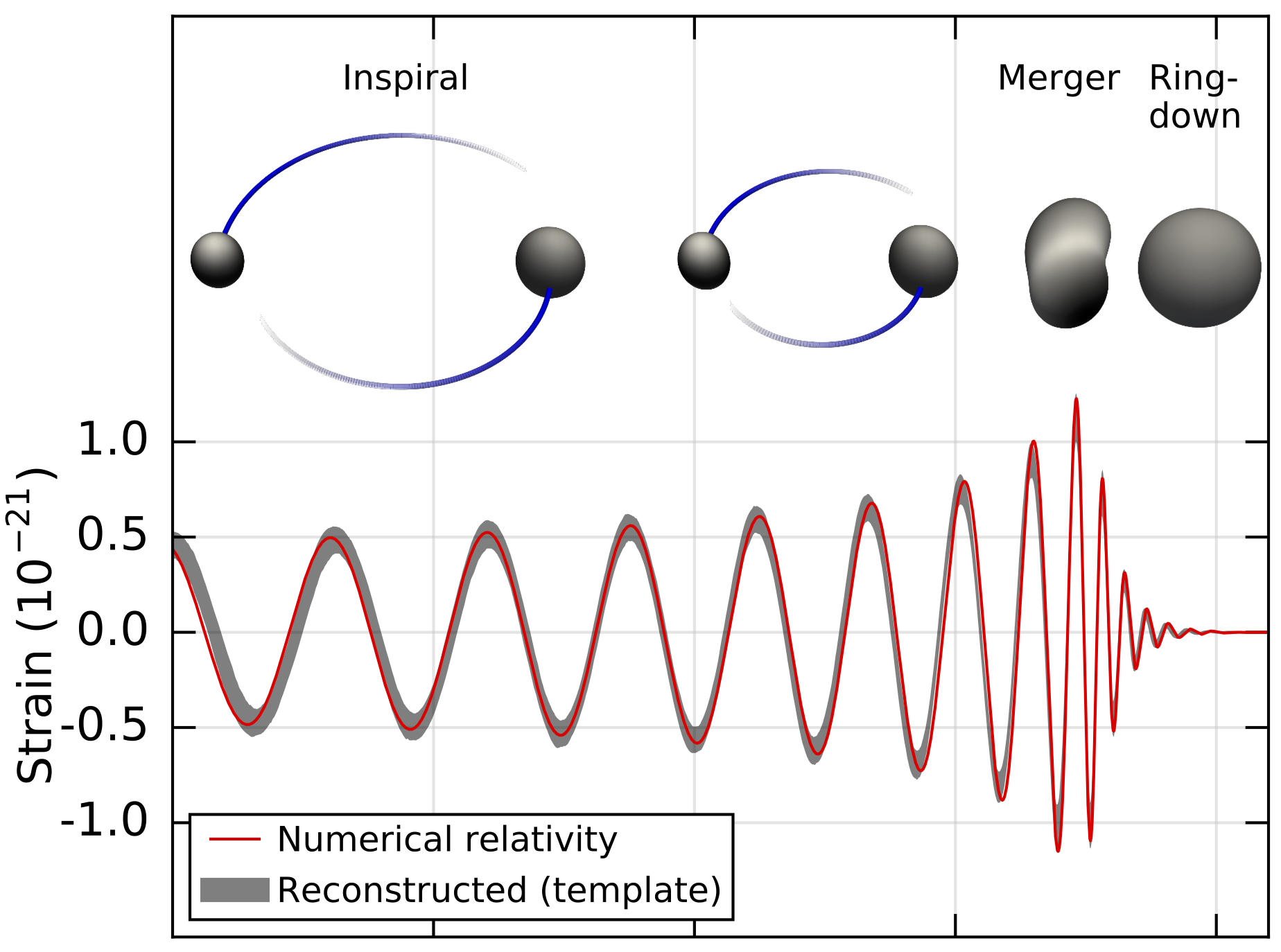
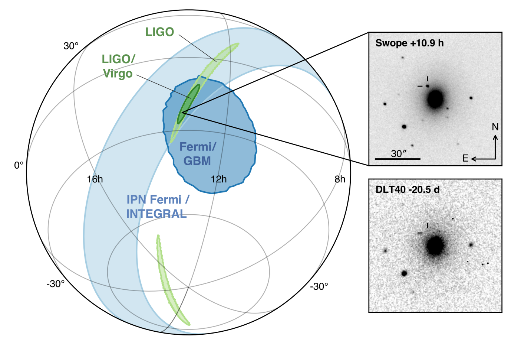
The Ghent Gravity Group focuses on the analysis of data coming from the LIGO, Virgo, and KAGRA experiments, and on developing data analysis techniques and a science case for the future GW detector network. The student would learn about the analysis of data, statistical methods, and astrophysics, and get involved with a large international collaboration in the active field of gravitational-wave science.
Project 3: Using galaxy clusters for cosmology with gravitational waves
One of the most important challenges of modern cosmology is the Hubble tension. It is a statistically significant difference between the values of the Hubble constant — the value describing the expansion rate of the universe — determined through different methods. We need a new method to measure the value of the Hubble constant and find out which one of the previous measurements is incorrect. Gravitational waves provide us a new and unique opportunity to measure this value and settle the Hubble tension. By observing the GWs from a merger of two compact objects (black holes or neutron stars), we can infer the distance of the source. If the same source emits electromagnetic radiation as well, then by finding its host galaxy, we can determine the redshift of the source. From the redshift and the distance, the Hubble constant can be inferred. So far, we have only observed a single source with GWs and EM radiation as well, the binary neutron star merger GW170817. However, even if we do not know the exact host galaxy, we can use a statistical method by taking into account all of the possible host galaxies. For that, we need a galaxy catalogue, such as the one used in the latest analyses, called GLADE+, the development of which was led by members of our group.
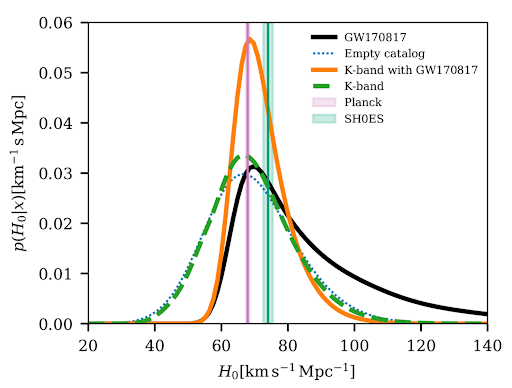
From May, the global network of GW detectors will operate again with unprecedented sensitivity. It means that GW detections will be more frequent than ever, and we can observe GWs from farther and farther away. Our galaxy catalogues are not yet 100% complete in these faraway regions of the universe, which leads to less accurate Hubble constant determinations. In this project, we try a brand new method to help GW cosmology: using galaxy cluster catalogues simultaneously with galaxy catalogues for the Hubble constant measurements. Cluster catalogues provide a less detailed view of the matter distribution in the universe, but they reach out to farther distances; hence, they can complement the currently used galaxy catalogues. A novel cluster catalogue with unprecedented depth and accuracy will be publicly released in the next couple of months, which makes this time absolutely ideal to start pioneering this technology.
Contact: Dr. Gergely Dálya
Project 4: Hunting down electromagnetic systematics for a gravitational-wave measurement of the Hubble constant
Compact binaries, i.e., binaries of black holes and / or neutron stars observed in gravitational waves (GWs) are self-calibrated distance indicators, or standard sirens. Together with a separate measurement of a redshift they can be used to measure cosmological parameters such as the Hubble constant H0, the local rate of expansion of the Universe. The value of H0 obtained from conventional late and early-universe probes have recently started diverging, a problem in cosmology known as the Hubble tension. An independent GW measurement of H0 can help resolve the Hubble tension and alter our view of modernism cosmology. The binary neutron star merger GW170817 together with redshift information from its optical counterpart already provided the first GW standard siren measurement of H0. The several binary black hole mergers together with electromagnetic information from galaxy catalogues help improve this measurement statistically [1]. It is expected that with several observations we obtain a H0 measurement competitive with conventional astronomy.
Since we are attempting a precise measurement, it is crucial to ensure accuracy. In other words it is important to study all possible sources of systematic errors which could otherwise bias the measurement, and develop a mechanism to correct for them.
In this project, we will look at various possible uncertainties on the galaxy catalogue side, including the galaxy luminosities, and their distributions. We will study how these uncertainties propagate, whether they leave behind any bias, and if so, how to correct for them. This work will play a central role in the measurement of H0 by the LIGO-Virgo-KAGRA Collaboration in its upcoming observing runs O4 and O5.
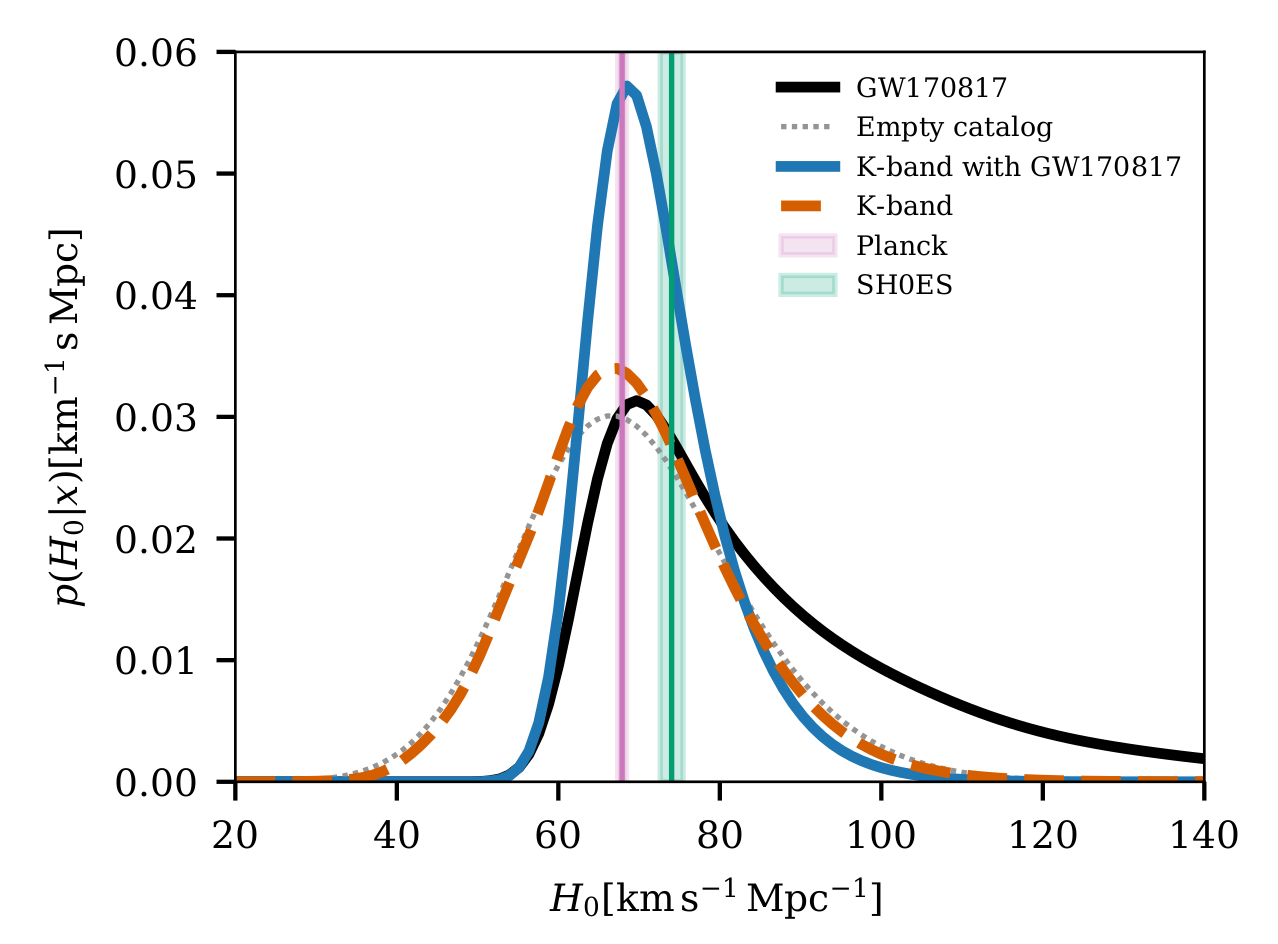

The student will be a member of the Virgo Collaboration. The work involved will be computational. The student will learn to use existing data analysis methods and develop new algorithms. The student will also learn about statistical methods, and get involved in a large international collaboration in the exciting field of GW science.
Contact: Cezary Turski
Project 5: Probing the large scale structure of the universe using gravitational-waves
The next generation of gravitational wave (GW) detectors such as the Einstein Telescope and the Cosmic Explorer will not only have an unprecedented sensitivity and distance reach, but will also lead to much better-localized compact binary sources than the current LIGO and Virgo detectors. As a result one would be able to map out the universe in its largest scales using GWs, just as it is done with electromagnetic (EM) probes. The large scale structure of the universe seen in GWs will carry information complementary to what is seen in EM astronomy. In particular, it will tell us about the properties of galaxies in the early universe, how binaries of black holes formed, and how long they took to merge.
In this project we will study the criteria that help improve the inference of the large scale structure of the universe using gravitational waves. Subsequently, we will map the inferred quantities (namely the “two-point correlation function”) to bounds on astrophysics.
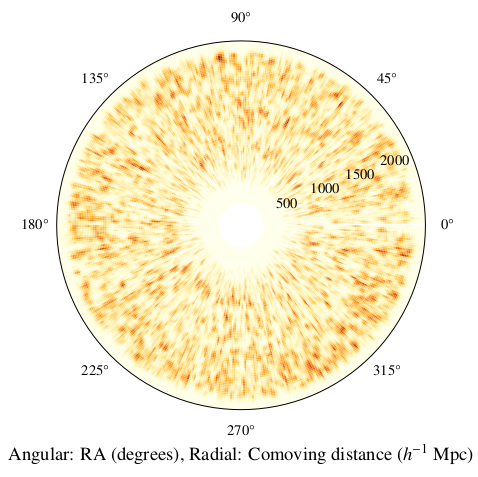
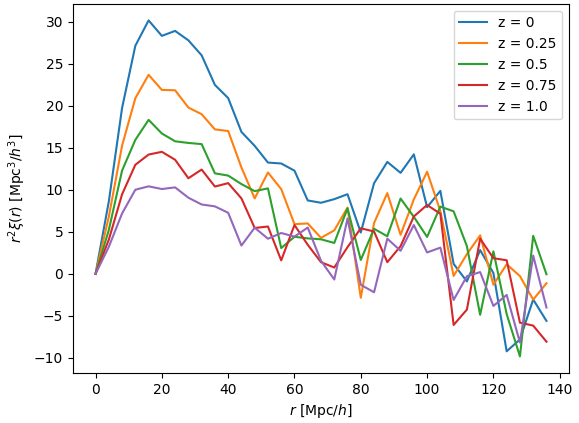
The student will be a member of the Einstein Telescope Collaboration. The work involved will be computational. The student will learn to use existing data analysis methods and develop new algorithms which will form the basis of data analysis techniques for the Einstein Telescope detector network. The student will also learn about statistical methods, and get involved in a large international collaboration in the exciting field of GW science.
Contact: Freija Beirnaert
Project 6: Searching for exotic compact objects using gravitational-waves
It is possible that the compact binaries observed by LIGO and Virgo are not binary black holes, but exotic compact objects mimicking black holes. Several such exotic compact objects have been proposed in literature [1]. These include boson stars, dark matter stars, gravastars held together by thin shells, or even quantum modifications to black holes such as fuzzballs and firewalls. A modification to the standard black hole geometry can lead to several observable effects in different regimes of the gravitational signal. Due to lack of knowledge about the exact nature of the exotic compact object, it may not be possible to use standard modelled search or parameter inference methods (such as matched filtering). On the other hand some features in the expected signal from even an unknown object can be expected to be robust.
In this project, we will bring together modelled and model-agnostic methods to optimally extract information about the nature of the merging compact binary. These analysis methods could help reveal unknown physics or could give us the first hints beyond Einstein’s general relativity.
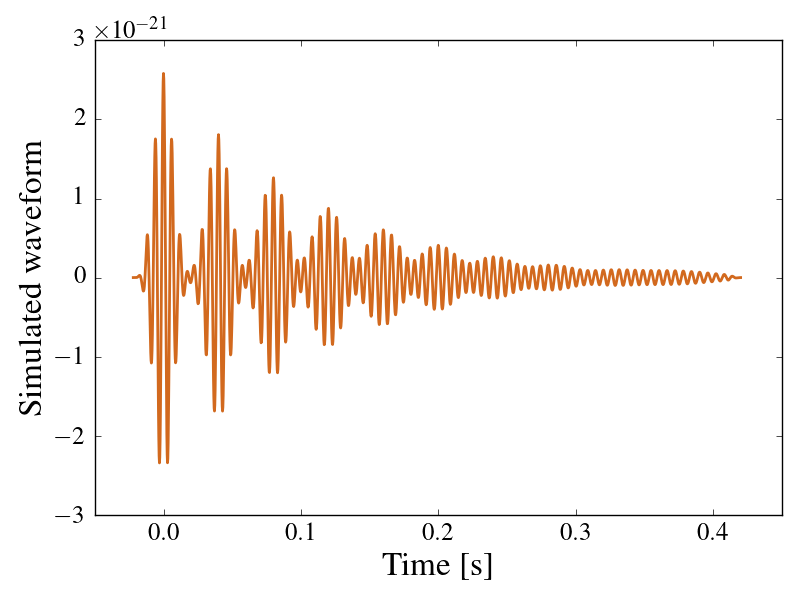
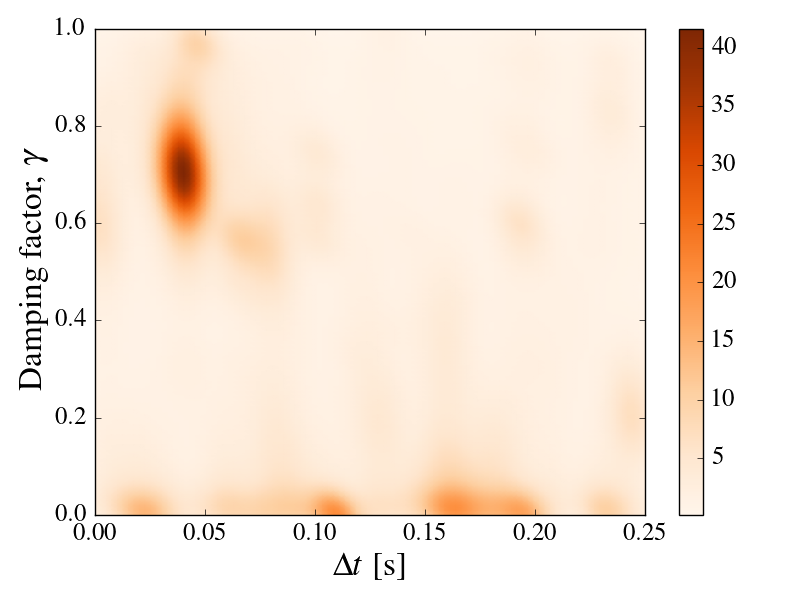
The student will be a member of the Virgo Collaboration. The work involved will be computational. The student will learn about statistical methods, get exposed to some existing data analysis methods, and will largely develop new algorithms as described above. The student will test the algorithm on existing LIGO-Virgo-KAGRA data as well as fresh data coming in during the O4 observing run. The developed codebase will eventually be used by the LIGO-Virgo-KAGRA Collaboration as a standard data analysis algorithm for testing general relativity and probing the nature of black holes. The student will be involved in a large international collaboration in the exciting field of GW science.
Contact: Prof. Archisman Ghosh